The global shift toward sustainable energy demands more than just incremental improvements—it requires bold rethinking of how we generate, store, and distribute power. While uranium-fueled nuclear reactors remain the standard, several lesser-known or experimental technologies offer the potential for safer, cleaner, and more efficient energy systems.
These concepts are not hypothetical. Most have been scientifically validated or demonstrated at small scales. What holds them back isn’t physics—it’s economics, infrastructure, or engineering complexity. But as the energy landscape evolves, these underutilised systems could become essential.
Below are nine advanced energy technologies that represent both the near future and the distant frontier.
1. Thorium Reactors
Thorium-based molten salt reactors (MSRs) have been discussed for decades—and for good reason. Thorium is abundant, cannot easily be weaponized, and produces significantly less nuclear waste. MSRs also operate at atmospheric pressure, minimising the risk of catastrophic failure.
Current limitations: Thorium cannot sustain a chain reaction on its own and requires a fissile seed like uranium-233. Most nuclear infrastructure is deeply rooted in uranium.
Outlook: Viable in the next few decades with focused investment, especially in nations like India and China that hold large thorium reserves.
2. Helium-3 Fusion
Fusion powered by helium-3 has attracted attention for its potential to produce energy without radioactive waste or neutron radiation. The main obstacle is sourcing: helium-3 is scarce on Earth but may exist in significant quantities on the Moon.
Current limitations: No fusion reactor—helium-3 or otherwise—has achieved net energy output. Lunar mining adds further complexity.
Outlook: High potential, but entirely dependent on breakthroughs in both fusion technology and space-based resource extraction.
3. Antimatter Reactors
Matter-antimatter annihilation is the most energy-dense reaction known. In theory, a few grams of antimatter could power a city. But practical use is hindered by the difficulty of generating and containing antimatter safely and efficiently.
Current limitations: Antimatter is produced only in microscopic quantities in particle accelerators. Storage requires advanced magnetic containment systems.
Outlook: Remains firmly in the realm of high-energy physics research, with limited near-term applications.
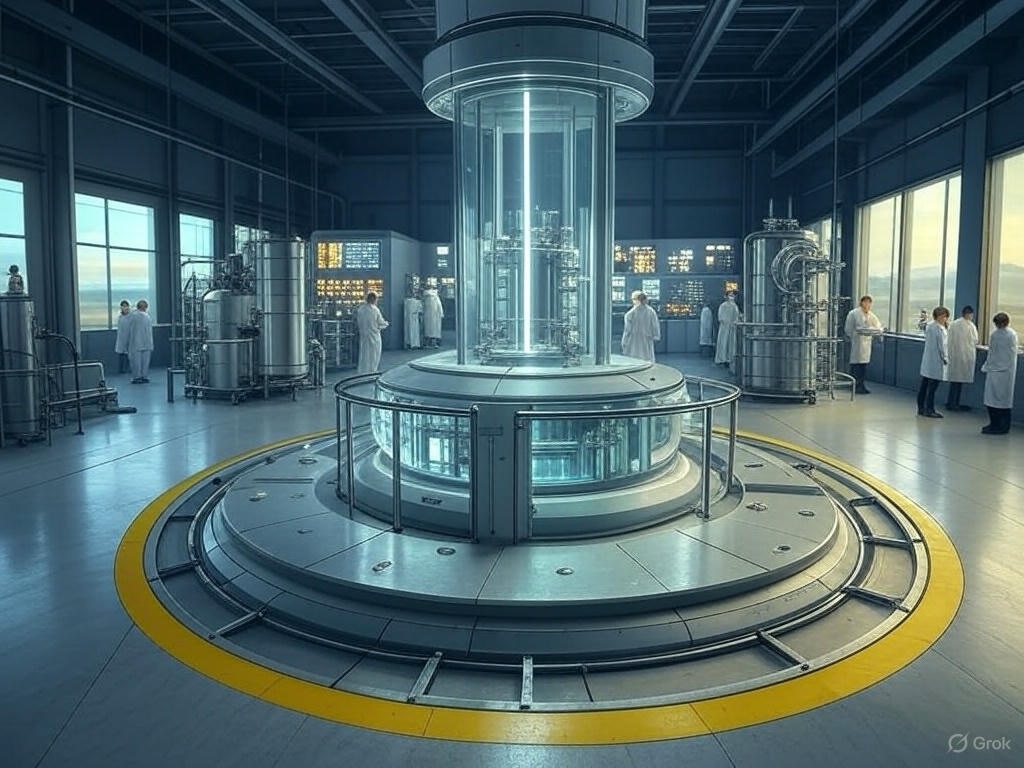
4. Pebble Bed Reactors
These small, modular reactors use ceramic-coated fuel pebbles and offer a passively safe design. If cooling fails, the reactor shuts itself down without melting down. Their high thermal efficiency and inherent safety make them attractive for distributed nuclear systems.
Current limitations: Manufacturing and handling of specialised fuel spheres remains complex. Deployment is limited to pilot programs.
Outlook: Promising for modular, scalable nuclear energy—particularly in regions seeking alternatives to large-scale plants.
5. Liquid Metal Fast Reactors
Using liquid metals such as sodium or lead as coolants allows these reactors to operate at higher temperatures with greater fuel efficiency. Some designs can even recycle spent nuclear fuel, significantly reducing long-term waste.
Current limitations: Liquid metals are reactive and challenging to manage, and most designs are still at prototype or research stages.
Outlook: Likely to play a role in next-generation nuclear systems focused on sustainability and waste minimization.
6. Muon-Catalyzed Fusion
This low-temperature fusion process uses muons to facilitate reactions without requiring extreme heat. While theoretically sound, muons decay rapidly, and generating them consumes more energy than the process currently yields.
Current limitations: Energy inefficiency and particle instability prevent practical application.
Outlook: Experimental. Could become viable with significant advancements in particle generation and control.
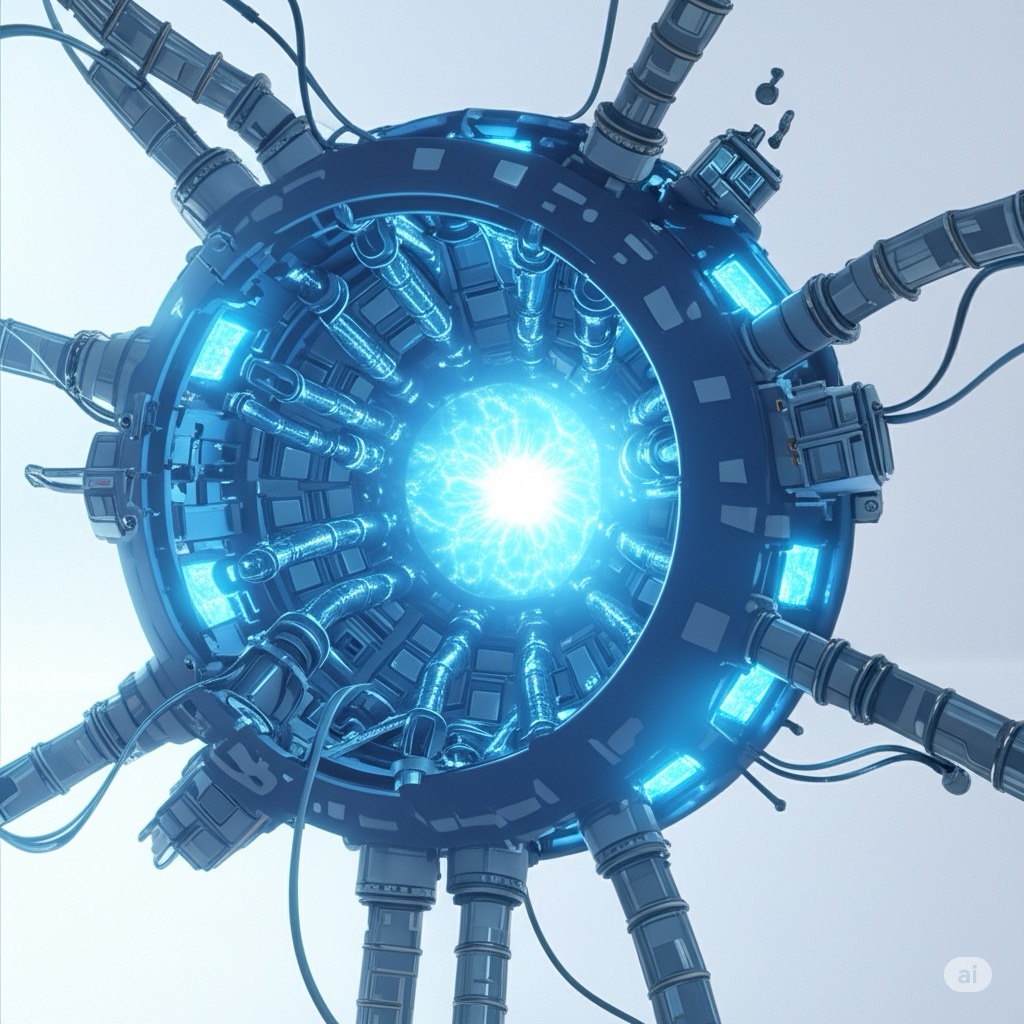
7. Z-Pinch Fusion
An older idea now undergoing a revival, Z-pinch systems use magnetic compression to force plasma into fusion conditions. While early models suffered from instability, modern materials and simulation tools may solve these challenges.
Current limitations: Maintaining stable plasma remains a core hurdle. No prototype has achieved sustained energy output.
Outlook: Worth watching. Several private firms are investing in compact Z-pinch reactors.
8. Betavoltaic Batteries
These nuclear batteries generate electricity using beta decay from isotopes like tritium. Already used in spacecraft and medical implants, they offer decades of low-level power without maintenance.
Current limitations: Low power density and regulatory hurdles limit civilian adoption.
Outlook: Ideal for long-duration, low-power applications where replacement is impractical—deep-sea, space, or embedded IoT devices.
9. LENR (Low-Energy Nuclear Reactions)
Popularized as “cold fusion,” LENR research has observed unexplained thermal anomalies at low temperatures. While controversial, some studies suggest possible nuclear-level energy release without ionizing radiation.
Current limitations: Lack of reproducibility and theoretical framework has kept LENR at the margins of mainstream science.
Outlook: Uncertain, but worth continued investigation given the potentially transformative implications.
Final Thoughts
While thorium reactors and liquid metal fast reactors may be closest to commercialization, more speculative systems like helium-3 fusion or antimatter power remain scientifically credible and technologically plausible—with the right breakthroughs.
In a world facing climate urgency and increasing energy demand, it’s not enough to invest in what’s easy. We need to explore what’s possible. These underused and emerging technologies offer more than incremental change—they hold the potential to redefine the boundaries of energy itself.
This blog post was written with the assistance of Copilot and ChatGPT, based on ideas and insights from Edgar Khachatryan. Pictures generated by Gemini and Grok,